Quantum Computing: The Next Frontier in Information Technology
Explore the revolutionary world of quantum computing, a cutting-edge technology that promises to transform information processing and problem-solving. Unlike classical computers that use bits, quantum computers harness the unique properties of qubits, enabling them to perform complex calculations at unprecedented speeds. Discover the fundamental principles of quantum mechanics, such as superposition entanglement, and how they empower quantum algorithms to outperform traditional systems in fields like cryptography, optimization, drug discovery, and materials science. Despite significant challenges like qubit stability and a shortage of skilled professionals, the future of quantum computing holds immense potential for enhancing artificial intelligence, data security, and technological innovations. Join the conversation on the implications of this groundbreaking technology for society and the digital landscape.
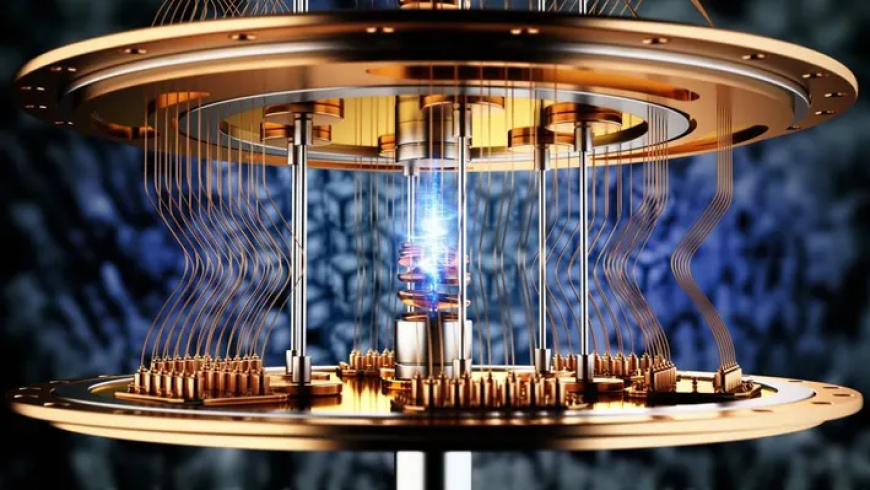
Introduction:
Quantum computing represents a revolutionary advancement in information technology that has the potential to redefine how we process, compute, and understand complex datasets. Building upon the principles of quantum mechanics, this innovative computing paradigm differs fundamentally from classical computing. While classical computers utilize bits as the smallest unit of data, which can exist in a state of 0 or 1, quantum computers leverage quantum bits or qubits. These qubits can exist in multiple states simultaneously, a property known as superposition, enabling quantum computers to solve problems that are currently intractable for classical systems. This essay delves into the fundamental principles of quantum computing, its potential applications, the challenges it faces, and its implications for the future of technology.
One of the most compelling features of quantum computing is entanglement, a phenomenon where qubits become interconnected in such a way that the state of one qubit can instantaneously affect the state of another, regardless of the distance separating them. This characteristic enhances the computational power of quantum systems, allowing them to perform complex calculations at unprecedented speeds. For instance, quantum algorithms like Shor's algorithm promise to factor large integers exponentially faster than the best-known classical algorithms, posing significant implications for fields such as cryptography.
Potential applications of quantum computing extend across various domains, including drug discovery, financial modeling, and optimization problems. In pharmaceuticals, quantum computers can simulate molecular interactions at a level of detail unattainable by classical computers, potentially accelerating the development of new medications. In finance, they can optimize portfolios and manage risk with greater accuracy, enabling more informed decision-making.
However, the path to practical quantum computing is fraught with challenges. Quantum coherence is delicate, and maintaining qubit stability over time is a significant hurdle. Additionally, error rates in quantum computations are currently high, necessitating the development of robust error-correction techniques. Researchers are actively exploring various quantum architectures, such as superconducting qubits and trapped ions, to overcome these obstacles and bring quantum computing closer to reality.
As we look to the future, the implications of quantum computing are profound. It not only has the potential to revolutionize industries but also to reshape our understanding of computation itself. As we stand on the brink of this new technological era, the collaboration between physicists, computer scientists, and industry leaders will be crucial in harnessing the full potential of quantum computing, paving the way for innovations that could redefine the boundaries of what is computationally possible.
Fundamental Principles of Quantum Computing
At the core of quantum computing lies the principle of quantum mechanics, particularly superposition and entanglement. Superposition allows qubits to be in a state of 0, 1, or both at the same time. This property vastly increases the computational power of quantum systems. For instance, while a classical system with just 10 bits can represent 2^10 or 1,024 different states, a quantum system with 10 qubits can simultaneously represent all 2^10 states, providing an exponential increase in processing potential.
Entanglement, another crucial principle, refers to the phenomenon where qubits become interconnected in such a way that the state of one qubit directly affects the state of another, regardless of the distance between them. This interconnectedness allows quantum computers to perform complex operations on multiple qubits simultaneously, further enhancing their computational capabilities. The ability to manipulate entangled qubits opens new avenues for parallel processing, enabling quantum systems to tackle problems that are currently intractable for classical computers.
Quantum algorithms such as Shor’s algorithm and Grover’s algorithm exemplify the unique advantages of quantum computing. Shor's algorithm, for instance, efficiently factors large numbers, a task that is computationally difficult for traditional computers, posing significant implications for cryptography. The potential to break widely used encryption schemes, such as RSA, underscores the urgent need for advancements in quantum-resistant cryptographic techniques. On the other hand, Grover's algorithm provides a quadratic speedup for unstructured search problems, demonstrating quantum computing's capacity to outperform classical systems in specific tasks. This capability is particularly relevant in fields such as database management and optimization problems, where rapid data retrieval is crucial.
Moreover, the principles of quantum computing extend beyond theoretical constructs. They are paving the way for practical applications in various domains, including drug discovery, materials science, and artificial intelligence. Quantum simulations can model molecular interactions with unprecedented accuracy, potentially revolutionizing the pharmaceutical industry. In artificial intelligence, quantum machine learning algorithms are being developed to enhance pattern recognition and data classification, promising to accelerate advancements in this rapidly evolving field.
As researchers continue to explore the depths of quantum mechanics, the convergence of theory and application in quantum computing heralds a transformative era in technology. The implications of harnessing these fundamental principles are profound, not only reshaping computing paradigms but also redefining our understanding of information processing in the universe. The journey into the quantum realm is just beginning, and its potential is limited only by our imagination and ingenuity.
Potential Applications of Quantum Computing
The applications of quantum computing span various fields, promising transformative advancements in science, technology, and problem-solving. In cryptography, quantum computers could render classical encryption methods obsolete by breaking widely used encryption schemes in polynomial time. This has led to the development of quantum-resistant cryptography, emphasizing the need for a secure digital landscape in the quantum era. As organizations increasingly rely on digital communications, the urgency for robust security measures becomes paramount. Quantum key distribution (QKD) offers a potential solution, allowing secure communication channels that are theoretically immune to eavesdropping.
In optimization problems, which are prevalent in industries such as logistics, finance, and manufacturing, quantum computing could significantly enhance decision-making processes. Quantum algorithms can efficiently explore multiple solutions simultaneously, optimizing routes, resource allocation, and investment strategies, ultimately leading to improved operational efficiency. For instance, companies in supply chain management could leverage quantum computing to minimize costs and delivery times, thereby gaining a competitive edge in the market. Financial institutions could also utilize quantum algorithms for risk assessment and portfolio optimization, enabling them to navigate the complexities of global markets with unprecedented precision.
Furthermore, quantum computing has exciting implications for drug discovery and materials science. By simulating complex molecular interactions at the quantum level, researchers can gain insights into new pharmaceuticals and materials, accelerating the development of innovative solutions in healthcare and technology. The ability to model and predict chemical reactions could lead to breakthroughs in personalized medicine, where treatments are tailored to individual genetic profiles. Additionally, the exploration of novel materials could revolutionize industries ranging from energy storage to electronics, paving the way for more efficient and sustainable technologies.
Moreover, the potential of quantum computing extends to artificial intelligence and machine learning. Quantum algorithms could enhance machine learning models by processing vast datasets more efficiently, leading to improved pattern recognition and predictive analytics. This capability could transform sectors such as healthcare, where predictive models can inform patient care, or autonomous systems, where real-time decision-making is critical.
Challenges in Quantum Computing Development
Despite its immense potential, quantum computing faces significant challenges that impede its widespread adoption. One of the primary hurdles is the issue of qubit stability and coherence. Quantum systems are highly sensitive to environmental disturbances, leading to decoherence, which disrupts the quantum states of qubits. Developing error-correcting codes and more stable qubit designs remains an active area of research. Researchers are exploring various materials and architectures, such as superconducting qubits and topological qubits, to enhance stability and mitigate the effects of noise.
Additionally, the scarcity of skilled professionals in quantum computing presents another formidable challenge. As this field evolves, the demand for a workforce proficient in quantum algorithms, quantum physics, and computer science is increasing. Educational institutions and research organizations must ramp up their efforts to train the next generation of quantum scientists and engineers. Initiatives such as specialized degree programs, online courses, and collaborative workshops can help bridge this skills gap. Furthermore, fostering interdisciplinary collaborations between physicists, computer scientists, and engineers is essential to accelerate innovation and application in quantum technologies.
Practical implementations also face technical barriers, such as the need for ultra-cold temperatures to maintain qubit coherence and the complexity of scaling quantum systems. Current quantum computers require sophisticated cryogenic setups to operate, which not only complicates their design but also increases costs. Addressing these technical factors is crucial for building commercially viable quantum computers. Researchers are investigating alternative approaches, such as room-temperature quantum computing and hybrid systems that integrate classical and quantum processors, to overcome these limitations.
Moreover, the integration of quantum computing into existing technological frameworks poses additional challenges. Developing robust quantum algorithms that can outperform classical counterparts in practical applications is a critical area of focus. Industries such as cryptography, pharmaceuticals, and materials science stand to benefit significantly from quantum advancements, yet translating theoretical potential into tangible solutions remains a complex endeavor.
While the path to quantum computing is fraught with challenges—ranging from qubit stability and workforce shortages to technical barriers and integration issues—the ongoing research and development efforts hold promise. By fostering education, innovation, and collaboration across disciplines, the quantum computing community can pave the way for breakthroughs that may one day revolutionize computation and problem-solving across various sectors.
Implications for the Future of Technology
As quantum computing matures, its implications for technology and society are profound. The predictive capabilities of quantum computers could lead to breakthroughs in artificial intelligence, enabling machines to learn and optimize more efficiently. By harnessing quantum algorithms, AI systems may process vast datasets at unprecedented speeds, revealing patterns and insights that were previously unattainable. This could revolutionize various sectors, including healthcare, where personalized medicine could be tailored to individual genetic profiles, or climate modeling, where complex simulations could yield more accurate predictions.
Furthermore, innovations in quantum communication can enhance data security, paving the way for the safe transmission of sensitive information. Quantum key distribution (QKD) allows for secure communication channels that are theoretically immune to eavesdropping, thereby protecting against cyber threats. This level of security is paramount in an era where data breaches and identity theft are rampant, making quantum communication a vital component of future cybersecurity strategies.
However, the rise of quantum computing also raises ethical and security concerns. The ability to break classical encryption poses significant risks to personal privacy and national security. As quantum computers become more accessible, the potential for malicious actors to exploit their capabilities increases. This creates an urgent need for organizations and governments to transition to quantum-resistant encryption methods to safeguard sensitive information against future threats.
As societies navigate these challenges, it is crucial to develop regulatory frameworks that address the ethical use of quantum technology. Policymakers must collaborate with technologists, ethicists, and industry leaders to establish guidelines that ensure responsible development and deployment of quantum innovations. This includes considering the implications of AI powered by quantum computing, which could amplify existing biases if not carefully monitored.
Moreover, fostering public awareness and understanding of quantum technology is essential to build trust and facilitate informed discourse. Education initiatives can empower individuals to engage with the ethical dilemmas posed by quantum advancements, promoting a more inclusive dialogue around technology's future.
Conclusion
Quantum computing represents a paradigm shift in computational capability that has the potential to revolutionize various domains, from cryptography and optimization to drug discovery. Despite facing challenges in qubit stability, workforce shortages, and practical implementations, the development of quantum technology continues to progress globally. As researchers unveil new quantum algorithms and improve system designs, we edge closer to realizing the transformative power of quantum computing. The implications for society and technology will be substantial, carrying both opportunities and challenges that require careful consideration and management. Thus, investing in research, education, and ethical frameworks will be vital as we stand on the brink of the quantum computing revolution.
What's Your Reaction?
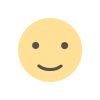
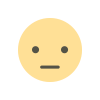
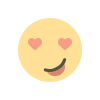
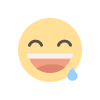
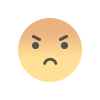
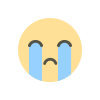
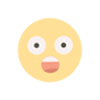